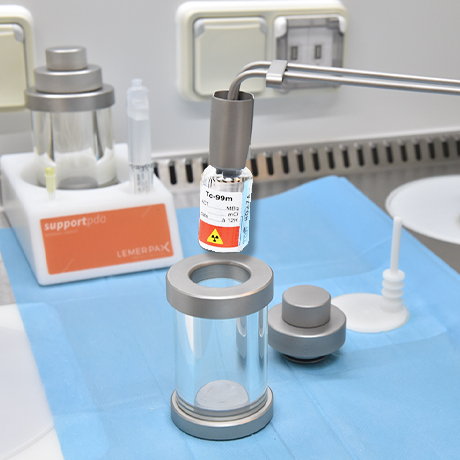
The Lifecycle of Radiopharmaceuticals: From Research to Clinical Use
Radiopharmaceuticals are drugs that contain radionuclides.
They are primarily used for two main purposes: diagnostic procedures and treatment assessments through imaging. The radiation detected by nuclear medicine cameras helps doctors observe and analyse how the human body functions.
Additionally, they serve therapeutic purposes, as the emitted radiation can specifically target cancerous or diseased cells. Although therapeutic use currently represents only about 10% of radiopharmaceutical applications, this practice is rapidly expanding.
Radiopharmaceuticals act as radiotracers and typically consist of two main components:
- The radionuclide: A radioactive isotope that emits radiation.
- The vector: A non-radioactive molecule that targets a specific organ, tissue, or cell.
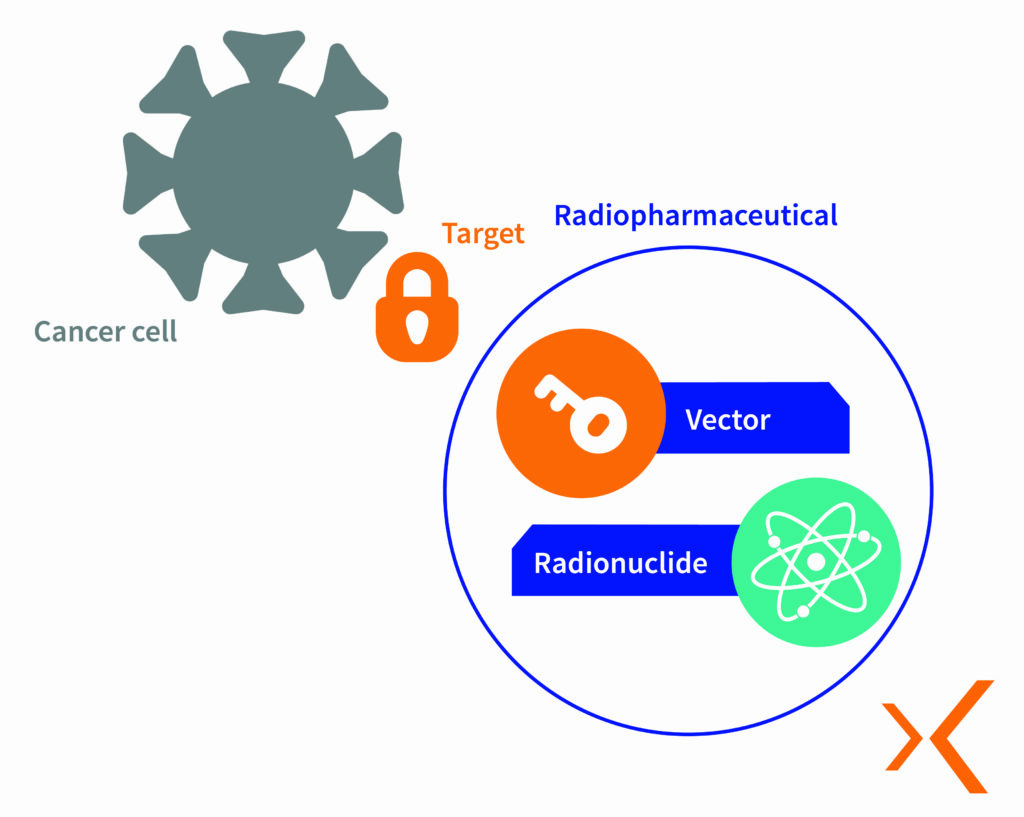
Radiopharmaceuticals contain radionuclides, also known as radioisotopes, which are tiny particles that emit radiation. Each radionuclide is carefully selected based on its stability, radioactive half-life, and emission characteristics required for medical applications. These radioisotopes are then combined with molecules chosen for their biological properties, which guide the radioisotope to the target cells where they bind to specific receptors.
New combinations are created with specific properties tailored for either diagnostic or therapeutic purposes, targeting precise areas of the body to deliver effective radiation. The lifecycle of radiopharmaceuticals, from research and development to clinical use, is complex and requires close collaboration between researchers, regulatory authorities, manufacturers, and healthcare professionals.
Innovation in the Lab: The Research and Development (R&D) Phase
The lifecycle of a radiopharmaceutical begins with research and development. This phase involves discovering and experimenting with new compounds, conducting preclinical studies to evaluate their toxicity and effectiveness, and performing clinical trials in humans to determine their medical utility.
The goal is to develop innovative associations or methods to enhance diagnostics and treatments, particularly for different types of cancer. In the next step, rigorous preclinical and clinical testing is conducted to ensure the purity, stability, and specificity of the compound.
These trials provide valuable insights into cancer mechanisms and patient responses to novel therapies. Only the most effective and reliable radiopharmaceuticals move forward to the next stage.
Regulatory Approval: Marketing Authorization (MA)
Once clinical trials are completed and data is collected, the radiopharmaceutical must be submitted to regulatory authorities for a thorough evaluation of its safety, efficacy, and quality. In France, this involves the National Agency for the Safety of Medicines (ANSM), while at the European level, the European Medicines Agency (EMA) is responsible. In the United States, the Food and Drug Administration (FDA) handles approvals.
Due to their radioactive nature, radiopharmaceuticals are subject to two sets of regulations: those governing pharmaceuticals (particularly potentially toxic substances) and those governing radionuclides. This dual regulatory framework involves the application of two sets of laws, regulations, and standards, along with specific requirements at each stage.
The new drugs must meet stringent criteria for:
- Quality: In line with pharmaceutical and analytical standards.
- Safety: In line with toxicological and pharmacological standards.
- Efficacy: In line with clinical standards.
Once approved, radiopharmaceuticals move into production and distribution.
Radiopharmaceuticals must comply with the same production standards as other drugs. However, their radioactive nature requires technological adaptations.
One key aspect is the lifespan of the products: each radionuclide has a specific half-life, the time it takes for the isotope’s radioactivity to decrease by half. The half-life can range from minutes to days or even thousands of years. For instance, a half-life of a few hours is ideal for medical imaging, allowing for quick diagnostic exams with limited radiation exposure. Conversely, therapeutic applications may require isotopes with longer half-lives to ensure prolonged treatment effects.
Most radiopharmaceuticals have a short radioactive half-life, which means:
- They must be manufactured frequently, either weekly or daily.
- Batch sizes are limited, ranging from 10 to 10,000 units.
- The production and distribution logistics must be carefully managed to meet the needs of nuclear medicine departments.
Throughout the entire production process, Good Manufacturing Practices (GMP) must be strictly followed to ensure production quality, protect patients, and, most importantly, guarantee the safety of workers, adhering to the ALARA* principle.
*ALARA : As low As reasonably Achievable, is one of the fundamental principles of protection against ionizing radiation.
Quality control measures are implemented to ensure the optimal performance of these drugs in clinical settings before they are administered to patients. Additionally, regulatory bodies such as the ASN (French Nuclear Safety Authority) and the IRSN (French Institute for Radiological Protection and Nuclear Safety) provide guidelines and recommendations.
There are two main methods for producing radiopharmaceuticals, depending on the nature of the product: ready-to-use radiopharmaceuticals and radiopharmaceuticals that require preparation using kits and generators
Ready-to-Use Radiopharmaceuticals
Ready-to-use radiopharmaceuticals are produced in a pharmaceutical facility. They are then transported to hospitals or examination centres. These are typically multi-dose medications, meaning that a single vial can be fractionated in the nuclear medicine department and then injected into multiple patients.
The Manufacturing Phases of Ready-to-Use Radiopharmaceuticals
First, the radionuclide is produced by irradiating a specific target within a research reactor or particle accelerators such as cyclotrons, causing a nuclear reaction.
The radionuclide is then purified to obtain the desired chemical form, usually as a salt or metal compound. This purification step is critical to ensure the quality and purity of the radionuclide used in the radiopharmaceutical.
The product from the cyclotron is collected in a shielded production hot cell in a radiopharmaceutical preparation area, which could be a hot lab or a clean room. Here, the radiosynthesis or radiolabelling phase begins: the radionuclide is combined with a specific vector to form the radiopharmaceutical.
The product is then transferred to shielded hot cells for dispensing and fractionation, where batches are prepared for use in nuclear medicine departments.
Using dispensing equipment and dosing stations, several operations are carried out to finalize the batches, such as:
- Labelling the batches
- Characterizing the activity concentration
- Performing necessary dilutions
- Preparing vials (filling, crimping, and transferring to lead shields or leaded vial holders).
Radiopharmaceuticals Reconstituted Using a “Cold” Kit
Radiopharmaceuticals that require preparation are manufactured in the hospital’s radiopharmacy unit, in shielded hot cells. Each dose is tailored to the patient’s specific needs.
Manual Constitution of Radiopharmaceuticals
Preparation kits contain a non-radioactive vector molecule designed to be labelled. To produce the final radiopharmaceutical, the labelling process is carried out in a shielded hot cell using a radionuclide generator.
Isotope generators contain the parent radionuclide, which decays to form a daughter radionuclide through a process such as elution.
This method is typically used for isotopes with a very short half-life. For instance, technetium-99m, the primary radioisotope used for low- and medium-energy radiodiagnostics, has a half-life of 6 hours and is generated from a molybdenum-99 source.
Another example, for high-energy applications, is gallium-68, which has a half-life of 68 minutes and is produced from the parent isotope germanium-68.
There are various labelling protocols depending on the radionuclides and/or the different organs and tissues to be explored.
The ready-to-inject patient dose is then packaged in a syringe shield until it is administered.
Once the radiopharmaceutical is prepared, the clock starts ticking
A radiolabelled drug must be prepared just a few hours or even minutes before use if its half-life is very short. This is why it’s sometimes necessary to have a production unit (such as a cyclotron) in hospitals or examination centres.
Clinical Use of Radiopharmaceuticals in Nuclear Medicine Departments
Clinical use marks the culmination of the radiopharmaceutical’s lifecycle. These drugs are typically administered to patients by injection as part of diagnostic or therapeutic procedures. The injection can be performed manually using syringe shields, or more commonly, with an automated shielded injector for optimal radiation protection.
Other methods of administering radiopharmaceuticals exist, such as oral administration (e.g., iodine-131 capsules) or inhalation (particularly using 99mTc or 133Xe).
In diagnostic imaging, radiopharmaceuticals are often used with imaging techniques such as gamma cameras, including Single Photon Emission Computed Tomography (SPECT) or Positron Emission Tomography (PET), providing invaluable functional imaging to detect complex conditions like cancers.
In therapy, radiopharmaceuticals are used to target and attack cancer cells or diseased tissues. They also pave the way for targeted therapies (RadioPharmaceutical Therapy), which allow for more precise treatments and fewer side effects commonly associated with traditional therapies like chemotherapy.
Continuous Monitoring of Radiopharmaceuticals with Pharmacovigilance
The main aim of pharmacovigilance is to monitor drugs to identify potential risks, report unforeseen side effects, and modify usage recommendations as necessary.
Pharmacovigilance ensures the safe and effective use of radiopharmaceuticals, helping improve the quality of care and protecting patient health.
Conclusion
The lifecycle of a radiopharmaceutical is a multifactorial process, with each stage being critical and essential for ensuring the safety and effectiveness of these drugs.
It is also a process of continuous innovation and improvement. Researchers are constantly exploring new compounds, refining synthesis techniques, and studying new radioisotopes with even more promising properties, ultimately enhancing patient care and outcomes.
This relentless pursuit of excellence aims to expand the capabilities of radiopharmaceutical products, thus opening new frontiers in medical imaging and therapy.